
Ever since humankind began to discern the overall structure of the solar system a few centuries ago, i.e., that the sun basically resides in the center and the planets orbit around it, this has brought forth questions, for example, how did the planets, and the solar system as a whole, form? A second question would be, are there other planetary systems in the galaxy, and if so, how common are they? To a pretty large extent, the answer to the second question depends upon the answer to the first, in that if the processes by which the planets in our solar system formed are those that would not be expected to happen very often, then planetary systems would accordingly be quite rare. If, on the other hand, these processes are commonplace, then so should planetary systems be common.
A theory in vogue during the early 20th Century proposed that the planets formed by a collision, or near-collision, between the sun and another star; the encounter pulled material out of the interior of the sun (and presumably the other star as well), and this material cooled down to form the planets. Since the distances between stars are such that collisions, or at the least very close encounters, between them would be expected to be very rare, then there are likely very few planetary systems; indeed, our sun and the other star involved might have the only ones. There were numerous issues with this idea, not the least of which is the unlikelihood that the hot material pulled out from the sun could cool down into planets rather than dissipate, and it fell out of favor within a few decades.
Another idea had been proposed in the mid-18th Century by the German philosopher Immanuel Kant, and then independently, and in greater detail, in the 1790s by the French scientist Pierre-Simon Laplace. In this “nebular hypothesis,” the sun was surrounded by an extended outer atmosphere, which due to rotation ended up being flattened into a surrounding disk. Over time the gaseous material in this disk cooled and solidified into the planets we see today. If this idea is correct then we might expect planetary systems to be rather commonplace, and during the late 19th and early 20th Centuries the “spiral nebulae” observed in telescopes were considered by some astronomers to be examples of this process. Today we know that these “spiral nebulae” are in fact external galaxies like our own, the spiral structures being composed of hot stars and gas.

There were nevertheless serious issues with this “nebular hypothesis,” including the fact that there seemed to be no efficient way that the material within this disk could condense into planets. In 1969 the Russian astronomer Viktor Safronov proposed what could be called a variation of the “nebular hypothesis” that ended up addressing many of these issues; these ideas were later expanded upon by the American astronomer George Wetherill, and while many of the details remain to be worked out, today these ideas are accepted as being generally correct.
The overall general picture that we have today of the planetary system formation process begins with an interstellar cloud of dust and gas. The shock waves from a nearby supernova explosion triggered the formation of condensations within this cloud, which under their own self-gravity began to coalesce into stars. As these stars continued collapsing their rotation rate began to increase, and this faster rotation caused a non-trivial amount of the material to fall into a surrounding disk instead of onto the star. Over time the dust in this disk began to stick together and accumulate, attracting still more dust and material until objects a few km in diameter, called “planetesimals,” were formed. In regions closer to the forming central star these planetesimals were primarily made of silicates and other “hard” material, whereas in the cooler regions far from the star the planetesimals were able to accumulate the gaseous, more “volatile” material as well.

Over timescales of tens to hundreds of millions of years, these planetesimals often collided with each other, and while the results of many of these collisions would involve the fragmenting of the planetesimals, some of these occurred at low enough relative velocities such that they stuck together, creating larger objects that, due to an increased gravitational attraction brought about by a greater mass, were able to collect still more planetesimals. Eventually, larger objects, called “protoplanets,” were formed, and the process continued, until eventually the large objects we today call “planets” resulted.
If such an idea is correct then we would expect to see evidence of such disks around other stars, especially younger ones where the planet-forming process is still going on. One of the earliest indications of such a disk came with the detection by University of California astronomer Martin Cohen in 1975 of the likely strong presence of water ice surrounding the young solar-type star HL Tauri. The presence of a gas disk was firmly detected at radio wavelengths by Annelia Sargent and Steve Beckwith in the mid-1980s, and has recently been imaged directly by the Atacama Large Millimeter/submillimeter Array (ALMA) telescope in Chile.
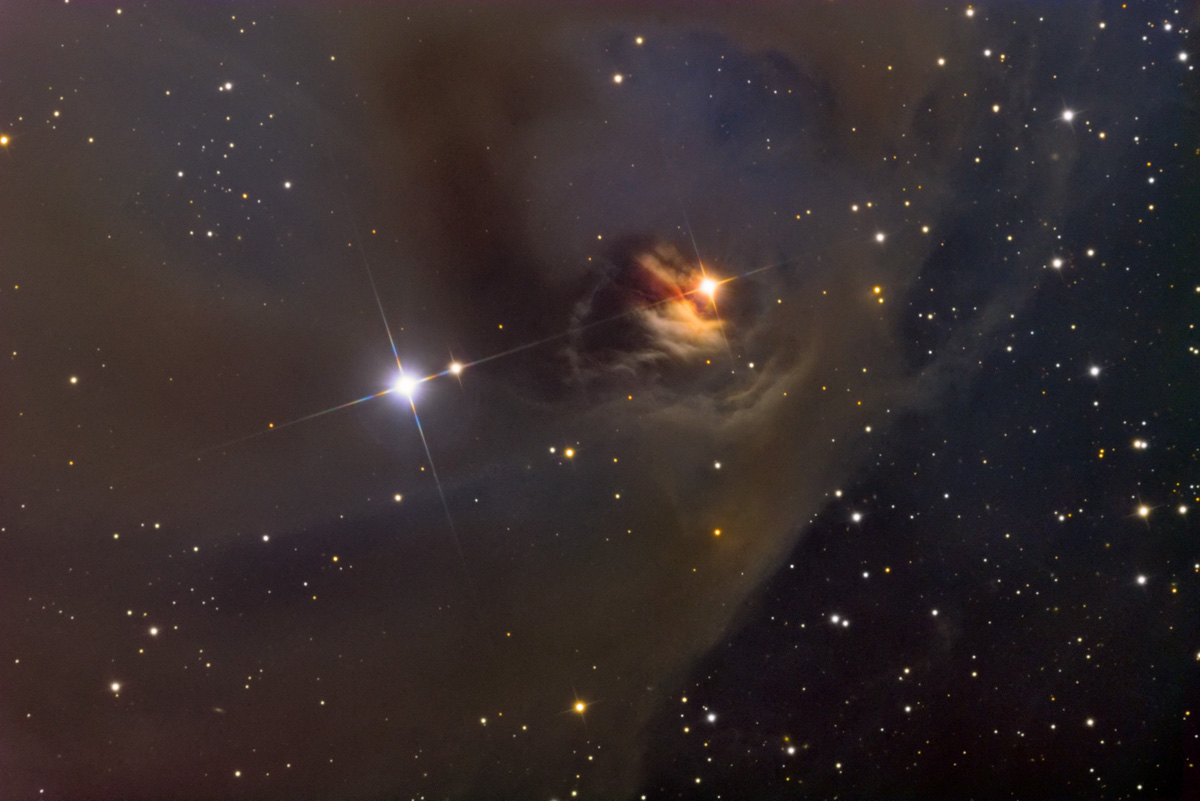
In 1983 the InfraRed Astronomical Satellite (IRAS) spacecraft detected excess infrared radiation, indicative of surrounding disks of dust, around several stars, including the bright naked-eye stars Vega and Fomalhaut. A year later, in December 1984 astronomers Brad Smith and Richard Terrile, utilizing telescope data taken from the Las Campanas Observatory in Chile, reported the first optical detection of such a disk, around the 4th-magnitude star Beta Pictoris. This particular star is of spectral class A6 and is approximately 1.75 times as massive as our sun; it is located 63 light-years away and is relatively young as stars go, being 20 to 26 million years old. The disk that Smith and Terrile detected extends over 1000 AU from the star and is presented nearly edge-on to our line of sight.
Numerous other such disks have been detected since then. The Hubble Space Telescope has detected several of these “proto-planetary disks,” including around stars located within the Orion Nebula M42. Numerous very young solar-like stars, called “T Tauri stars” after the prototype (and which exhibit irregular flares of brightness, hence their classification as variable stars), exhibit spectral and/or other evidence for the presence of such disks; some of the older T Tauri stars do not show such evidence, suggesting that the planet formation process has essentially been completed around these objects.
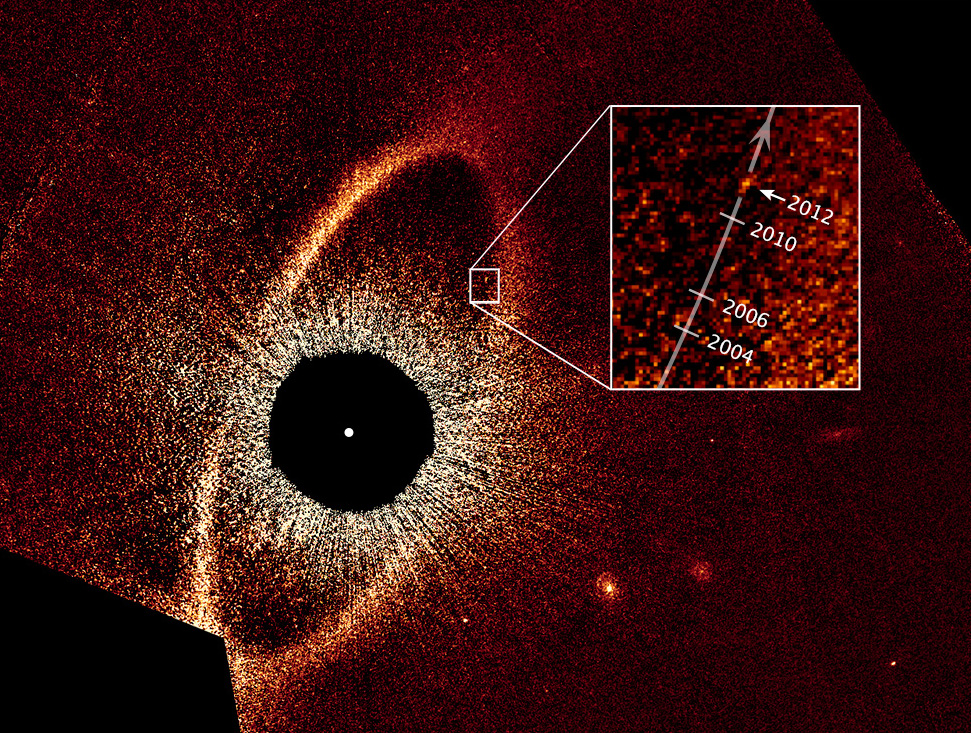
The disk around Beta Pictoris has been especially well studied ever since the announcement of its discovery in 1984. It exhibits rotation as well as a distinct amount of structure including various sub-regions, rings of material, and a slight warping at its edges. At least one planet has been found orbiting within the disk, being perhaps seven times as massive as Jupiter and orbiting at a distance of 9 AU. The existence of a possible second planet, of similar mass but closer to the star, was announced in 2019 and confirmed just a few months ago, and meanwhile evidence for possible “exocomets” was reported in the mid-1980s and the existence of these was confirmed in early 2019, as discussed in a previous “Special Topics” presentation.
Similar forms of evidence have now been detected in and around the disks surrounding various other stars, as well as planets in some cases. A distant planet orbiting within the disk surrounding the bright star Fomalhaut was reported twelve years ago, although whether or not this object is an actual “planet” has recently been questioned; meanwhile, a handful of planets have been detected orbiting around T Tauri stars. Overall, at this writing over 4350 planets have now been confirmed orbiting around other stars, with over 700 multiple-planet systems having now been found. The evidence is overwhelming that planetary systems are indeed very commonplace in the Galaxy – indeed, it would appear that many, if not most or even almost all, stars have accompanying planets – and that the basic processes by which the planets in our solar system formed operate throughout the Galaxy as well. While the planets we have discovered thus far rather strongly suggest that our solar system is not a typical planetary system – and in fact there appears to be no such thing as a “typical” planetary system – the basic underlying processes are still there.

These processes are not necessarily clean and orderly, however. Indeed, it is quite clear that the early solar system was a rather violent place, since there were many more planetesimals and protoplanets around then than there are today. The current model for the formation of the moon involves a Mars-size protoplanet – that has been given the name Theia – that was likely orbiting near one of the Earth-sun L4 or L5 Lagrangian points (discussed in a previous “Special Topics” presentation) that eventually was perturbed away from that spot into a collision path with Earth over four billion years ago. The moon subsequently would have coalesced out of the combined debris from Earth and from Theia. (Although versions of this model had been around for some time, it started to become the favorite in the late 1980s as a means of explaining the orbital and rotational configuration within the Earth-moon system as well as the internal geological structure of the moon and the composition of moon rocks returned by the Apollo missions.)
Although the consensus about this is not universal, there is relatively strong evidence that a period of “Late Heavy Bombardment” occurred in the inner solar system between 4.1 and 3.8 billion years ago. As mentioned previously, the population of planetesimals in the inner solar system during that era was significantly larger than it is today, and it is evident that the moon sustained many of the large impacts that produced the “maria” we see today during that time. The geological consequences for Earth during this time would have been significant, and since life was getting started on Earth during that same time frame the biological consequences may have been even more profound.

To some extent, things seem to be much more quieter now, although “quieter” is perhaps a relative term. The discovery of the “hot Jupiter” planets – large massive planets orbiting very closely to their respective parent stars – back in the mid- to late 1990s introduced the idea of “migration” to our models of the formation and evolution of planetary systems, and it is quite clear now that the planets, including – especially – the large ones like Jupiter and Saturn, have migrated their orbital distances over the lifetime of the solar system. Over very long timescales, i.e., hundreds of millions to billions of years, none of the orbital configurations are stable, and the planets will undergo migration in the distant future. The effects on the other objects in the solar system, including Earth, would be enormous; for example, in one set of scenarios, Mercury could be perturbed out of its present orbit and undergo a close encounter with Venus, after which several possibilities could ensue, including an impact by Mercury into Earth.
While all this is going on, the sun will be undergoing its own evolution, eventually to reach the “red giant” phase some five to seven billion years from now, and it is far more likely that Earth will become uninhabitable due to changes in the sun than that it will become uninhabitable as a result of an impact by Mercury. For now, our solar system remains the relatively quiet place that we see it as being today . . . but nothing lasts forever.
More from Week 52:
This Week in History Comet of the Week Free PDF Download Glossary
Ice and Stone 2020 Home Page