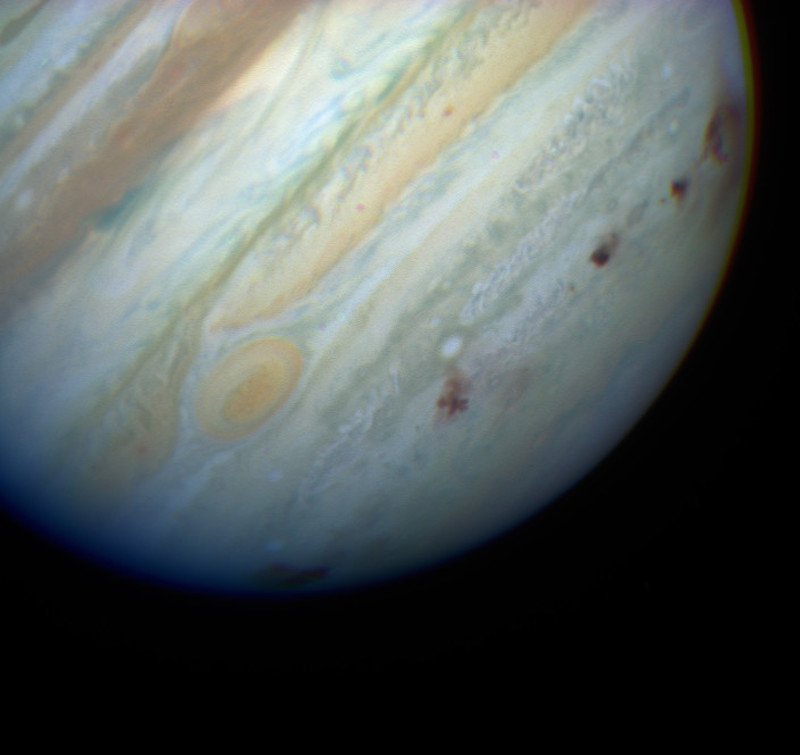
During the third week of July 1994 the various fragments of Comet Shoemaker-Levy 9 1993e impacted Jupiter, liberating enormous amounts of energy in the process and creating large planet-sized “scars” in Jupiter’s atmosphere that lingered for some time afterwards. (Comet Shoemaker-Levy 9 and these events are discussed in a future “Comet of the Week” presentation.) The events provided a vivid demonstration of the destructive power contained within such impacts, and with the thought that “it could be us next time,” people on Earth started to give serious thoughts as to how to prevent such things from happening here. The U.S. Congress chartered a special commission, headed by renowned planetary geologist and Comet Shoemaker-Levy 9 co-discoverer Eugene Shoemaker, to investigate this issue and report on the best ways to address it, particularly focusing on the identification of threatening objects.
The Shoemaker Commission delivered its report to Congress, and to NASA, in June 1995. The bulk of the report describes ways to implement comprehensive survey programs, given that the state of imaging technology had by that time reached the point where such programs were possible. With programs as they described, it would be possible to identify 90% of all threatening objects 1 km or larger in diameter within the next ten years. The survey programs that began coming on-line within the next few years, and which are described in a future “Special Topics” presentation, came about in no small part due to the recommendations of the Shoemaker Commission.
The primary rationale, certainly, is to identify any threatening objects before they might have an opportunity to threaten us. If a potentially threatening object could be identified two or three decades in advance, it should then be possible to do something about it; while destruction may not be feasible (or necessarily even advisable), a small change in its orbital motion 30 years in advance could make a significant difference in its location at the time of its closest approach, and if such a change could be made when an object is near aphelion and thus is exhibiting its slowest orbital motion, this would in turn require the lowest amount of energy. Specific deflection strategies that could be employed are the subject of a future “Special Topics” presentation.
The key, then, is not only to discover potentially threatening objects, but to determine their orbits with sufficient accuracy so that not only are we able to identify those that might threaten us someday, but also allow us to send spacecraft missions to them if that becomes necessary. Doing this requires weeks and months of follow-up observations, which may not be as glamorous as discovery but which is almost every bit as important. If the orbit can become well enough known so that the object in question can be recovered during a subsequent visit to near-Earth space, or identified in older images taken during a previous visit, this helps greatly in refining the orbit still further.
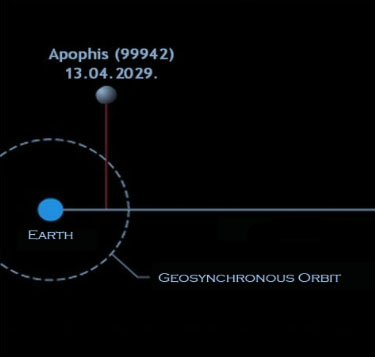
The practice of determining positions of objects such that orbits can be calculated is called “astrometry,” and since these are measured against the positions of background stars, good catalogs of stars’ positions are necessary. (Fortunately, with spacecraft-measured stellar positions being determined by ESA’s Hipparcos mission in the early 1990s and the present ESA Gaia mission, such catalogs are now available.) In general, the more observations – and, in particular, the longer the orbital arc covered by those observations – the better when it comes to determining accurate and precise orbits.
If the sun and the orbiting object were the only objects around, the calculation of its orbit and the determination of its location at future times would be a simple and straightforward matter. However, there are other objects, like Earth and other planets (especially Jupiter for objects out that far), and each of these gravitationally influences the objects’ orbits. Mathematically, while the “two-body problem” is relatively easy to solve, it turns out that there is no analytical solution possible for the “three-body problem” or the general “n-body problem,” and the best that can be achieved is a numerical solution that approximates the true orbit. Fortunately, with modern computer technology high-accuracy numerical solutions are achievable without too much difficulty.
Additional effects can come into play for certain types of objects. Small asteroids, particularly those which can best be described as loose piles of dust, can be subject to effects produced by solar radiation such as the Poynting-Robertson effect which causes a slow decrease in an object’s angular momentum. Comet nuclei can experience the effects of erupting jets off their surfaces, a phenomenon described as “non-gravitational forces” and described in a previous “Special Topics” presentation. Objects that pass near the sun during the course of their orbits can experience the effects of General Relativity.
When all of these considerations are allowed for, the process of calculating orbits, and predicting future approaches to Earth, commences . . .
On March 11, 1988 the IAU’s Central Bureau for Astronomical Telegrams created a stir when it issued IAU Circular 6837, about the asteroid 1997 XF11 (now permanently designated as (35396)). This object had been discovered the previous December by Jim Scotti with the Spacewatch survey based in Arizona, and orbital calculations based upon a three-month arc indicated that, on October 26, 2028, 1997 XF11 would be passing just 0.00031 AU from Earth – just barely over six Earth radii above the surface, close enough to be of significant concern given the uncertainties still involved. Within a day images of 1997 XF11 from photographs taken in 1990 were identified, and the now eight-year-long arc moved the “miss distance” in 2028 to a much more comfortable 0.0062 AU. The accurate determination of its orbit was helped still further when it passed 0.064 AU from Earth in October 2002 and was extensively observed, including by me.
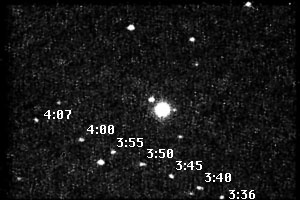
This entire episode involving (35396) 1997 XF11 has helped in refining how forthcoming close approaches are treated – and in how they are presented to the general public. What has happened on several occasions is that a recently-discovered object will be described as having a “1 in X” chance of impacting Earth at some future date; what is really happening is that, at that future date, the uncertainties in the object’s orbit will create a volume of space that it could theoretically occupy at that time, called its “error ellipsoid,” and if Earth happens to be within that “error ellipsoid” the probability of any impact is simply the volume of Earth divided by the volume of that ellipsoid. As additional observations come in and the object’s orbit gets better defined, the odds of an impact may actually increase, but this is entirely due to the fact that the “error ellipsoid” shrinks in volume, while Earth’s volume, of course, remains the same. Eventually, the “error ellipsoid” will shrink to the point that Earth is no longer inside it, and the probability of impact drops to zero.
The most dramatic example of this process involves the asteroid now known as (99942) Apophis. This object was discovered in June 2004 but only observed for two nights, until accidentally re-discovered that following December. Calculations soon began to show the possibility of an impact on April 13, 2029, and as per the usual occurrences the odds of an impact kept increasing; however, instead of suddenly dropping to zero, the odds kept increasing until they were as high as 1 in 40 before the possibility of an impact vanished. The “miss distance” in 2029 is now firmly established as being 0.00026 AU, or 31,000 km (five Earth radii) above the surface – within the orbital distance of geosynchronous satellites. Apophis is roughly 400 meters in diameter and should be a naked-eye object of 3rd magnitude when it flies by Earth; this event will be best seen from the eastern hemisphere. This remains the closest predicted future approach of any known asteroid. (For what it’s worth, the encounter date – April 13 – is a Friday.)
Even though an Earth impact by Apophis in 2029 has been completely ruled out, for a while it seemed possible that Apophis could impact Earth seven years later, on April 13, 2036. The 2029 approach is close enough that gravitational perturbations by Earth – which would be strongly dependent on the actual distance between the two objects – could place Apophis on an intercept trajectory if it were to pass through a certain window, or “keyhole,” 800 meters wide during that approach. This possibility has now been ruled out, and the 2036 “miss distance” is a relatively large 0.14 AU, on April 4. At this time there is still a very tiny chance (odds 1 in 150,000) of an impact in April 2068.
There are several organizations around the world dedicated to keeping track of any potentially threatening objects. The IAU’s Minor Planet Center is charged with announcing discoveries of asteroids and comets, collecting observations, and publishing orbits. The Center for Near Earth Object Studies is run by NASA to maintain information about potentially threatening objects, and the Near Earth Objects – Dynamic Site performs a similar function for ESA. The Spaceguard Foundation is a private organization based in Italy that acts as somewhat of a clearinghouse for near-Earth object observation efforts and information, and in fact the entire collection of worldwide observatories engaged in this effort is sometimes referred to as “Spaceguard,” a name taken from Arthur C. Clarke’s 1973 novel “Rendezvous with Rama” as an homage. Another private organization, the B612 Foundation based in California, is primarily devoted to developing deflection techniques but is also involved in searching for threatening objects.
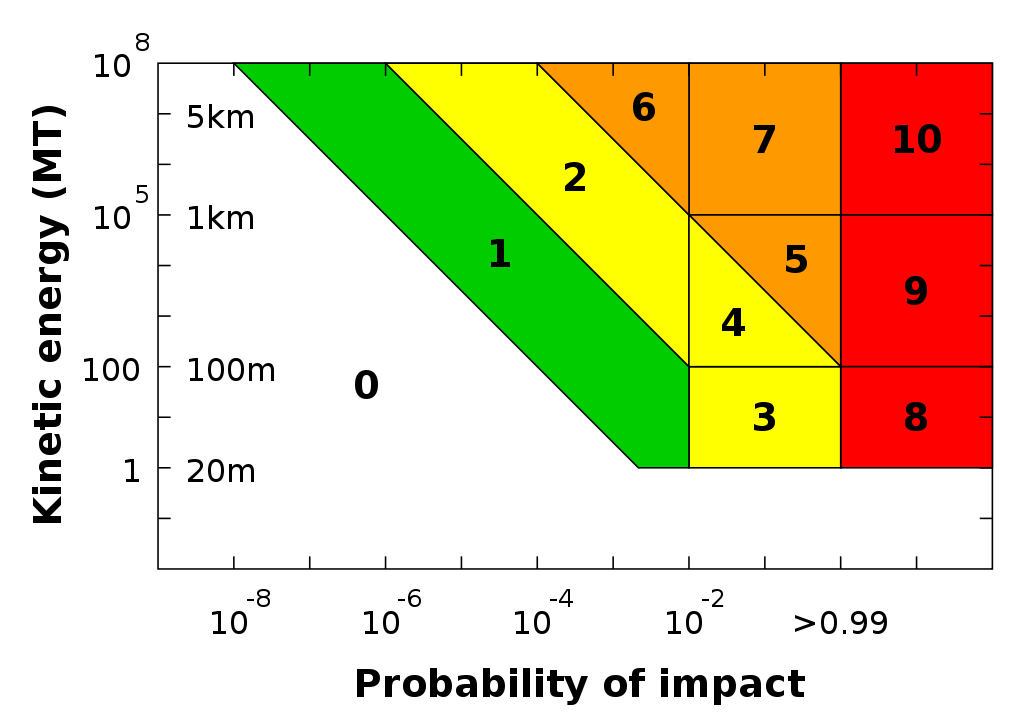
Although its usage is not strictly formal, many scientists in the near-Earth object community utilize the Torino Scale, developed by MIT astronomer Richard Binzel, as a means of numerically quantifying the risks of potentially threatening objects. The Torino Scale uses integers from 0 to 10, with 0 meaning no chance of an impact and 10 meaning a definite impact of global catastrophe, with allowances being made for both the calculated odds of an impact and the size of the potentially impacting object (and thus the kinetic energy an impact would release). Since its introduction a handful of newly-discovered objects have briefly rated a “1” on the Torino Scale before being dropped to “0;” the only object to receive a higher rating was Apophis, which reached the level of “4” during the brief period when calculations were indicating a close to 3% possibility of an impact in 2029. At this time no objects are listed as being higher than “0.”
The color codes on the Torino Scale are:
White (level “0”): no hazard
Green (level “1”): normal [no cause for public concern]
Yellow (levels “2,” “3,” and “4”): meriting attention by astronomers
Orange (levels “5,” “6,” and “7”): threatening
Red (levels “8,” “9,” and “10”): certain collisions
More from Week 16:
This Week in History Comet of the Week Free PDF Download Glossary
Ice and Stone 2020 Home Page